The belief that when you die, your soul sticks to the nearest refrigerator. Nov 06, 2019 Magnetism is defined as an attractive and repulsive phenomenon produced by a moving electric charge. The affected region around a moving charge consists of both an electric field and a magnetic field. The most familiar example of magnetism is a bar magnet, which is attracted to a magnetic field and can attract or repel other magnets. Teach children about the invisible power of magnetism with the 4M Magnet Science Kit. The Magnet Science Kit teaches young scientists about the effects of magnetism through a combination of fun science experiments and games. The kit contains materials to make interactive magnetic objects like the Super Power Horseshoe Magnet and the Magnet Wand.
Magnetism
Magnets are objects, which can attract, or pull, on some metals, like iron and steel. If you rub a piece of steel with a strong magnet, the piece of steel will because a magnet too. It has become magnetized. Other metals, like copper or gold, are not attracted to magnets. Magnets can also attract each other, but only if they face in opposite directions. A magnet has two ends called poles; one end is the north pole and the other is the south pole. A north pole will attract a south pole; the magnets pull on each other. But the two north poles will push each other away. We say the magnets repel each other. Magnets seem to act something like positive and negative electric charges. Electricity and magnetism are very closely related.
If the words north and south remind you of anything, the earth is in fact a giant magnet. A compass is a tiny magnet balanced on a point so it can turn freely. The magnet is attracted by the earth’s magnetic north pole and always points in that direction.
A good way to see how the magnet attracts is to do the following experiment. Take a strong bar magnet and putt a piece of pare over it. Then sprinkle some iron filings on the paper. The iron fillings will make a pattern. This pattern shows the magnetic field. The drawing below shows what the magnetic field looks like for a bar magnet (although the lines don’t really exist). Now try it with two magnets. Point their north poles at each other. The iron filings show how the magnetic field looks when the magnets repel. Now turn one magnet in the other direction to see how the magnets attract.
Discussion
history
Outline of the story told historically. Basic ideas that even young children now know. Keep everything to an introductory level.
- There are rocks that attract other rocks, but only if they're of the right kind
- These rocks will try to align themselves north-south (roughly speaking)
First comes Thales of Miletus (635–543 BCE) Greece (Ionia). Miletus is now on the western coast of Turkey in what was then a region of Greece known as Ionia (source of the chemical term ion, but that's another story).
- The stones of Magnesia (μαγνήτης λίθος)
- Μαγνησία now Manisa, Turkey
- Magnetite. A piece of magnetite with an exceptionally strong magnetic action is sometimes called a lodestone.
A nice quote from Thales would be nice here.
Some minerals such as magnetite (Fe3O4) are obviously magnetic.
Chinese navigators knew that magnetic rocks align themselves north-south (the south-pointing spoon).
The compass in real sense was created by a Chinese geomantic omen master in late Tang Dynasty, who originally used it for divination.
Find something historical.
The north magnetic pole of a compass points in the general direction of the north geographic pole of the Earth. Since opposite magnetic poles attract, this means that the south magnetic pole of the Earth is somewhere near its north geographic pole.
Animal Magnetism Osrs
Next comes Peter Peregrinus (as he is known in English) a.k.a. Pierre Pèlerin de Maricourt (presumably his proper french name) a.k.a. Petrus Peregrinus de Maharncuria (his latin title, which means 'Peter the Pilgrim of Maricourt'). Peter wrote what is commonly known as the Epistole de Magnete or Letter on the Magnet. It's full title is Epistola Petri Peregrini de Maricourt ad Sygerum de Foucaucourt, militem, de magnete (Letter on the Magnet of Peter Peregrinus of Maricourt to Sygerus of Foucaucourt, Soldier'). It was written on 8 August 1269 during the siege of the city of Lucera — the last remaining stronghold of Islam on the 'calf muscle' of the boot-shaped peninsula that is now called Italy.
Peter's work was so complete that no further studies on the properties of magnets were done until the monumental work of William Gilbert in 1600 — Tractatus sive Physiologia Nova de Magnete, Magneticisque Corporibus, et Magno Magnete Tellure (On the Magnet, Magnetic Bodies, and the Great Magnet of the Earth). De Magnete was the text in which Gilbert revealed the results of his research on magnetism and attempted to explain the nature of magnets and the five motions associated with magnetic phenomena. The work met with great acclaim and was republished in 1628 and 1633.
William Gilbert (1544–1603) England
Find something.
fundamentals
magnetic elements
- The big three
- iron (Fe)
- nickel (Ni)
- cobalt (Co)
- plus the oddballs
- gadolinium (Gd)
- dysprosium (Dy)
More on ferromagnetism later
The Earth is a magnet, geomagnetism, poles: north seeking and south seeking
rule of action: opposite poles attract, like poles repel
Magnetism & Electricitymr. Mac's Class 2019 2020
types of magnets
- permanent
- induced
- electromagnet
magnetic field
informal definition: compare to the other fields
phenomena | origin | field | symbol | ||
---|---|---|---|---|---|
gravity | force due to | mass | force per | mass | g |
electricity | force due to | charge | force per | charge | E |
magnetism | force due to | poles?* | force per | pole?* | B |
the real definition appears later
dilemma: breaking a magnet, there is no magnetic monopole, even down on the atomic scale there is no magnetic monopole, field lines heal themselves
Still, even though we don't have a formal definition of the magnetic field there's no reason why an informal definition can't suite us for a while.
properties of magnetic field lines
- direction determined from the north pole of a compass
- strength proportional to the density of the field lines
- field lines are closed loops
- field lines penetrate magnetic materials (compare this to electric field lines, which terminate on the surface of conductors)
- field lines bend at the surface of a magnetic material
The symbol for the magnetic field is B (boldface) when describing the full vector quantity and B (italic) when describing the magnitude alone.
The SI unit of the magnetic field is the tesla [T], named in honor of the Serbian-American electrical engineer Nikola Tesla (1856–1943) born in a part of the Austro-Hungarian or Hapsburg Empire that is now the independent nation of Croatia. Tesla was a pioneer in the associated disciplines of alternating electric current and rotating magnetic fields. His basic designs for electric motors, generators and transformers in the early part of the 20th century were little changed by the beginning of the 21st century.
Another unit in common usage is the gauss [G], named in honor of the German mathematician Carl Friedrich Gauss (1777–1855). Gauss is generally regarded as the greatest mathematician of all time. Of particular interest to physicists were Gauss' work on curved surfaces, which were important in the fields of electrostatics and general relativity (all puns intended). The gauss is also a unit in the cgs system that was originally developed by Gauss and is sometimes also known as the Gaussian system.
Each of these units will be defined formally in a later section of this book. Right now I will tell you that the tesla is the bigger unit.
1 T = 10,000 G
The tesla is in fact too big for most practical purposes. As such it is usually divided into microtesla [μT] or nanotesla [nT]. The gauss is also a bit too large, but not as bad as the tesla, so milligauss [mG] and microgauss [μG] are more common.
1 T | = | 10 kG |
1 mT | = | 10 G |
1 μT | = | 10 mG |
1 nT | = | 10 μG |
The following table lists the magnetic field strength for various devices, events, or phenomena. Whenever possible a location was also specified. Like the gravitational and electric fields, the magnetic field grows smaller with increasing distance form the source.
B (T) | location, event |
---|---|
1013 | neutron star, theoretical upper limit |
1010–1011 | neutron star, magnetar |
108–109 | neutron star, radio pulsar |
1000 | highest laboratory field, ephemeral |
100 | white dwarf star |
45 | highest laboratory field, sustained |
16 | strong enough to levitate frogs |
13 | strongest superconducting magnet |
2.4 | strongest permanent magnet |
1–4 | MRI |
1 | strong laboratory magnet |
0.45 | large sunspot |
0.15 | iron bar magnet, at poles |
0.10 | refrigerator magnet |
0.001 | Sun, at poles |
400 × 10−6 | Jupiter, surface mean |
100 × 10−6 | Sun, surface mean |
60 × 10−6 | Earth, at poles |
45 × 10−6 | Earth, surface mean |
30 × 10−6 | Earth, on equator |
10 × 10−6 | AM radio broadcast at receiver |
1 × 10−6 | solar radiation on Earth's surface |
180 × 10−9 | 100 W light bulb at 1 m, peak |
150 × 10−9 | Mercury, surface mean |
150 × 10−9 | Earth, altitude of geosynchronous orbit |
50 × 10−9 | Earth, magnetosphere nose |
35 × 10−9 | Moon, surface |
5 × 10−9 | interplanetary space near Earth |
1 × 10−9 | Earth, magnetosphere tail |
500 × 10−12 | interstellar space |
100 × 10−12 | intergalactic space |
50 × 10−12 | human heart |
100 × 10−15 | human brain |
ferromagnetism
This section is intended to be a discussion of magnetism on the small scale, not just the magnetism of iron, nickel, and cobalt (although that will be its primary focus). Perhaps it should be titled 'micromagnetism'.
Everything's due to electron spin. Well, almost everything.
type | spin alignment | examples |
---|---|---|
ferromagnetic | all spins align parallel to one another | iron, cobalt, nickel, gadolinium, dysprosium, heusler alloys |
ferrimagnetic | most spins parallel to one another, some spins antiparallel | magnetite (Fe3O4), yttrium iron garnet (YIG) |
antiferromagnetic | periodic parallel-antiparallel spin distribution | chromium, FeMn, NiO |
paramagnetic | spins tend to align parallel to an external magnetic field | oxygen, sodium, aluminum, calcium, uranium |
diamagnetic | spins tend to align antiparallel to an external magnetic field | nitrogen, copper, silver, gold, water, organic compounds |
superdiamagnetic | all spins align antiparallel to an external field | meissner effect in superconductors |
alloys
Steel…
type | composition | magnetic? | other characteristics |
---|---|---|---|
ferritic stainless | Fe, Cr, C | yes | moderate corrosion resistance, moderate durability |
austenitic stainless | Fe, Cr, Ni, C | no | high corrosion resistance, moderate durability |
martensitic stainless | Fe, Cr, C | yes | moderate corrosion resistance, high durability |
nonstainless (high carbon) | Fe, C | yes | low corrosion resistance, high durability |
Alloys made expressly for permanent magnets…
- Alnico
- Samarium alloys
- Neodymium alloys
Ferromagnetic alloys made entirely of nonferrous metals…
- Heusler alloy: copper, tin, manganese in the ratio 2:1:1. Friedrich Heusler (1866–1947) Germany. The copper may be replaced by silver. The tin may be replaced by aluminum, arsenic, antimony, bismuth, or boron.
- CrO2
- EuO
magnetic recording
the basic mechanism
media formats
- analog
- audio
- video
- digital
media shapes
- wire recording
- reel
- drums
- disks
substrates
- flexible: tape and floppy disks, typically polyester
- rigid: hard drives, typically aluminum
ferromagnetic material
- hard vs. soft
- Hard magnetic materials require relatively strong magnetic fields to become permanently magnetized and to reverse or erase the magnetization. They are most appropriate for digital data storage
- Soft magnetic media require relatively weak magnetic fields to become magnetized. They are more appropriate for analog audio and video recording.
type | bias | material | comments |
---|---|---|---|
I | normal | gamma ferric oxide (γ-Fe2O3) | first commercially manufactured in 1937 |
II | high | chromium dioxide (CrO2) | later replaced by layers of ferric oxide (Fe2O3) and cobalt (Co) with similar magnetic characteristics |
III | ferric chrome (FeCr) | quickly became obsolete | |
IV | metal | finely ground metallic iron | later replaced by mixtures of finely ground iron and cobalt |
n/a | barium ferrite (BaFe12O19) | magnetic stripes on bank and credit cards, high coercivity, less susceptible to accidental erasure |
transition temperatures
The Curie temperature is named for the French physicist Pierre Curie (1859–1906), who discovered the laws that relate some magnetic properties to change in temperature in 1895.
The antiferromagnetic equivalent of the Curie Temperature is called the Néel Temperature in honor of the French physicist Louis Néel (1904–2000), who successfully explained antiferromagnetism in 1936.
elements | TC (K) |
---|---|
iron | 1043 |
cobalt | 1404 |
nickel | 628 |
gadolinium | 289 |
erbium | 32 |
dysprosium | 155 |
ferrous compounds | TC (K) |
barium ferrite | 720 |
strontium ferrite | 720 |
Alnico | 1160 |
Alumel | 436 |
Mutamel | 659 |
Permalloy | 869 |
Trafoperm | 1027 |
NdFeB | 580 |
SmCo5 | 990 |
Sm2Co17 | 1070 |
nonferrous compounds | TC (K) |
CrO2 | 390 |
CuAlMn3 | ? |
LaxCa1−xB6 | 900 |
MnAs | 318 |
MnBi | 633 |
MnSb | 587 |
polymerized C60 | ~500 |
material | TN (K) |
---|---|
CoCl2 | 25 |
CoF2 | 38 |
CoO | 291 |
chromium | 475 |
Cr2O3 | 307 |
erbium | 80 |
FeCl2 | 70 |
FeF2 | 79–90 |
FeO | 198 |
FeMn | 490 |
α-Fe2O3 | 953 |
MnF2 | 72–75 |
MnO | 122 |
MnSe | 173 |
MnTe | 310–323 |
NiCl2 | 50 |
NiF2 | 78–83 |
NiFeO | 180 |
NiO | 533–650 |
TiCl3 | 100 |
UCu5 | 15 |
V2O3 | 170 |
animal magnetism (magnetotaxis?)
- creepy crawlies
- magnetotactic bacteria
- flatworms (Platyhelminthes)
- honey bees?
- fish
- chinook salmon (Oncorhynchus tshawytscha)
- yellowfin tuna (Thunnus albacares)
- amphibians
- red-spotted newt (Notophthalmus viridescens viridescens)
- reptiles
- loggerhead sea turtle (Caretta caretta)? or is it some other variety ?
- birds
- rock pigeon (Columba livia)
- bobolink (Dolichonyx oryzivorus)
- mammals
- naked mole rat (Heterocephalus glaber)
- Siberian hamster (Phodopus sungorus) ? or is it some other variety ?
- brown bat (Eptesicus fuscus)
health and safety
text
device | B (μT) |
---|---|
color tv/computer crt display | 500 |
electric stove | 1000 |
hair dryer | 1000 |
maglev train | 100 |
text
location | median (μT) | range (μT) | |
---|---|---|---|
earth's surface | 45 | 40–60 | |
workplace: | clerical worker w/o computer | 0.05 | 0.02–0.20 |
clerical worker w/computer | 0.12 | 0.05–0.45 | |
machinist | 0.19 | 0.06–2.76 | |
electrical line worker | 0.25 | 0.05–3.48 | |
electrician | 0.54 | 0.08–3.40 | |
welder | 0.82 | 0.17–9.60 | |
home: | typical US home | 0.09 | 0.03–0.37 |
MRI
magnetic resonance imaging (nuclear magnetic resonance)
- atoms in a magnetic field will absorb and then release energy as radio waves
- each atom that's visible to MRI has its own radio frequency (each atom is its own radio tower)
- All nuclei that contain odd numbers of nucleons have an intrinsic magnetic moment and angular momentum
- What atoms can MRI see (nmr frequencies at 1 T)?
- (42.38 MHz) hydrogen 1
- (40.05 MHz) fluorine 19 (in a lot of medications)
- (16.33 MHz) lithium 7 (not a lot in our body, but found in drugs for treating bipolar disorder)
- sodium 23 (used by neurons to make electric signals)
- (17.25 MHz) phosphorous 31 (involved in energy containing compounds)
- (10.71 MHz) carbon 13
- potassium (used by neurons to make electric signals)
- The same atoms in different molecules absorb and emit radio waves at slightly different frequencies
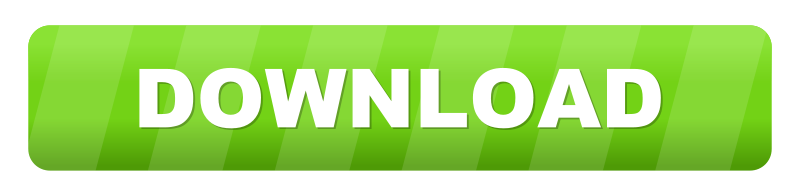